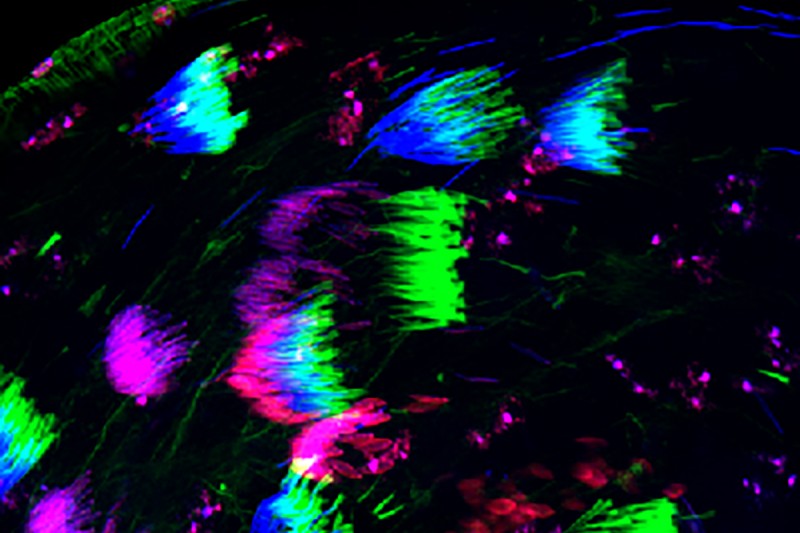
The lab of developmental biologist Dr. Eric Lai has been studying evolutionary battles of the sexes in fruit flies. This colorful snapshot, courtesy of the Lai Lab, depicts sperm maturation in fruit fly testes.
Developmental biologist Eric Lai, PhD, and his lab at Memorial Sloan Kettering Cancer Center (MSK) have been studying hotbeds of biological conflict.
Only instead of battles between outside invaders and internal defenders, they’ve uncovered an evolutionary battle of the sexes fought using a group of recently emerged genes. This conflict between the X and Y chromosomes plays out in a hidden struggle between “selfish” genetic actors and the small strands of RNA that keep them in check.
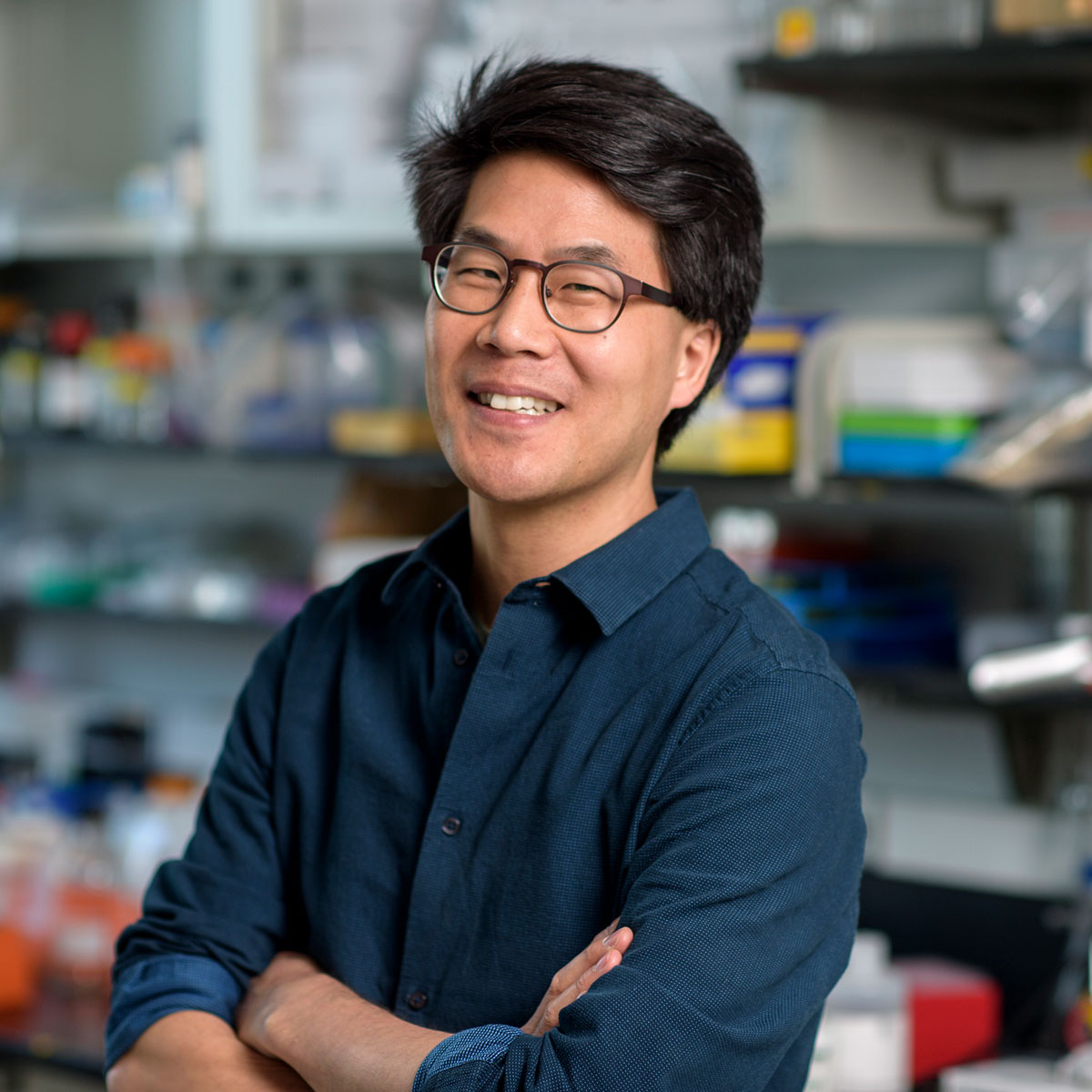
MSK developmental biologist Dr. Eric Lai
The common wisdom is that genes are preserved from generation to generation because they help the organism survive and reproduce. But Dr. Lai’s team is showing — in fruit flies — that certain genes can threaten a species’ very survival by pursuing their narrow self-interests in passing along their own genetic material. And this threat has given rise to molecular countermeasures to deactivate these rogue genes.
In a conversation that ranges from Shakespeare to Lewis Carroll to Wile E. Coyote, Dr. Lai, an investigator at MSK’s Sloan Kettering Institute, explains the research and its significance — and why it’s happening at a cancer center in the first place.
The Lai Lab’s latest findings on the topic were recently published in PLOS Biology and PLOS Genetics.
This idea of an organism needing to protect itself from its own genes is a bit counterintuitive, isn’t it?
Exactly! The concept is both confusing and surprising. Normally, we think that genes exist in our DNA because they encode something beneficial, helping us to develop from a fertilized egg to an adult, and ultimately allowing us to have children. But it turns out there are actually some “bad” genes that do not benefit the organism. Instead, their normal activity is, selfishly, to force their own transmission through the germline — that is, into the cells that carry the genetic information that is passed down from parents to children. This process is called “meiotic drive,” and the action of such selfish genes can come at a great cost to the host. In theory, they might even cause an entire species to go extinct.
In that way, they’re reminiscent of other selfish factors, like transposons (DNA sequences that can jump around in the genome) and viruses — whose main goal is to make more copies of themselves and spread those copies through the population. Obviously, in the past few years, we’ve become painfully aware of how harmful such entities can be, like the virus that underlies COVID-19. It’s just not much appreciated that there are genes that basically function in a similar way.
We’re studying this phenomenon in fruit flies, which have a short life cycle and for which we have great tools to conduct genetic manipulation. This has made them one of the most important models over the past century to learn about how genes work, especially during development and related to specific behaviors.
If the bad genes are part of the genome, how does the body recognize them as bad?
That question is what makes these genes very hard to study!
Generally speaking, we have many defense mechanisms against transposons and viruses. Several key protectors come in the form of small regulatory RNAs, which are collectively only 20 to 30 nucleotides (or letters of the genetic code) long. We found that a special class of these, termed small interfering RNAs (siRNAs), are harnessed to silence selfish meiotic drive genes via the RNA interference (RNAi) pathway.
How does this work? Most of the body’s defense mechanisms work by distinguishing between self and not-self. And there are parts of viruses and transposons that look decidedly foreign.
So the question here is, how does the body suppress selfish genetic elements that appear to be a normal part of the genome?
To make an antidote to these problem genes — and quickly, before the species is in trouble — we think that a piece of the gene gets copied over somewhere else in the genome, where it starts to make these siRNAs. These have a complementary genetic sequence to the problematic genes and trigger their silencing.
What happens when these bad actors aren’t silenced by the RNAs?
Our research shows that these selfish genes want nothing more than to unfairly pass along to the next generation — even though it’s disastrous for the organism as a whole to have such biased genetic inheritance. The “drive” genes we are studying are all located on the X chromosome. In many animals, including flies and humans, females have two X chromosomes, while males have an X and a Y chromosome. Incredibly, these selfish genes prevent the Y chromosome sperm from maturing. In this way an affected father can only have daughters — meaning that X-linked gene gets forced into all the kids!
Now, while this is very good from the perspective of the self-serving gene, it’s very bad for the population because you start running out of males really quickly.
If that’s not strange enough, we even found genes that, when they’re not silenced by protective siRNAs, cause affected fathers to be completely infertile. We liken this to a biological enactment of the line in Hamlet: “For ’tis the sport to have the enginer / Hoist with his own petard.” Shakespeare meant this as an ironic outcome when a military combatant sets a bomb — a petard —that blows up in their face. A more juvenile analogy might be Wile E. Coyote, who tries to hunt down the Road Runner with rocket skates or a bomb, but in the end simply hurts himself. In this genetic battle within the fly testis, if these selfish genes were permitted to function at full tilt, they might cause the species to go extinct!
It’s plausible that events like this may have eliminated species in the past — but without these organisms and their DNA available to study, we can’t know for sure. We believe this is probably happening all the time, and the species that are still around are the ones that have figured out a way to silence these problematic genes.
How did you get interested in this line of research?
We’ve been studying the production and biology of small RNAs for a long time. This line of research originated 15 years ago, when we discovered genes that produce siRNAs in the fruit fly species Drosophila melanogaster. Logically, these should silence something, so we looked for evidence of their targets. However, flies that completely lacked all siRNAs seemed to be largely OK, at least in our lab tests.
The key insight came when we started to look in other species of flies beyond Drosophila melanogaster, which is the most common species used in research. Active sex chromosome conflicts were known in a very closely related species, Drosophila simulans — though their molecular and regulatory bases were poorly understood.
We made D. simulans mutants lacking siRNAs that we speculated to be involved in controlling sex chromosome conflicts. Indeed, removing these suppressor siRNAs from the genome caused the levels of these selfish gene products to skyrocket and have these harmful impacts on male reproduction.
Now, D. simulans is a very successful species of fly. This species originated around Madagascar only about 250,000 years ago, but since then has spread all over the planet, going wherever humans went. It seems like they’re thriving — but genetically they’re at tremendous risk. Who could guess that such a successful species is kind of hanging by a thread, trying to keep internal genetic troublemakers at bay?
What do the lab’s two new papers show?
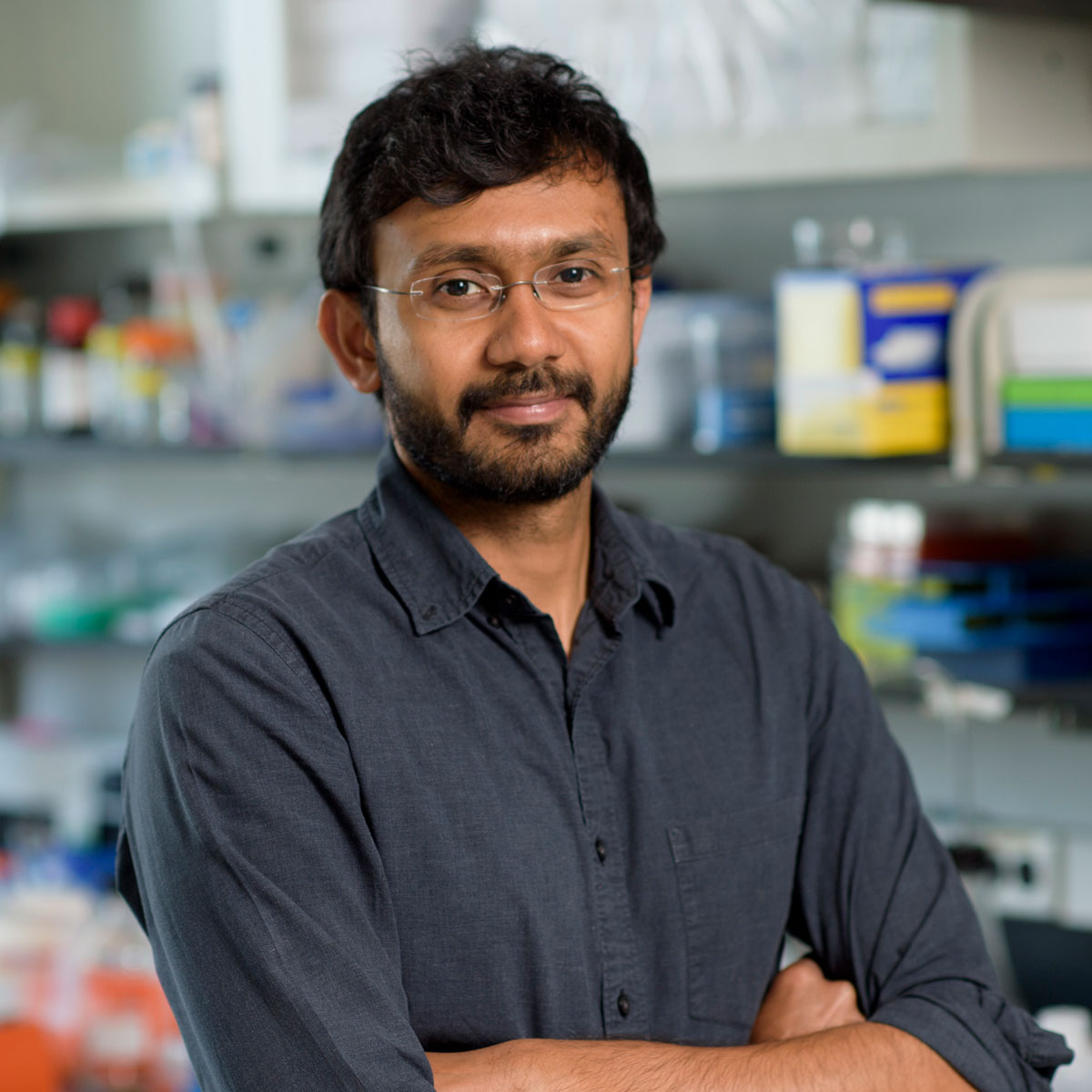
Dr. Jeffrey Vedanayagam
Both of the recent papers were led by postdoctoral fellow Jeffrey Vedanayagam, PhD, from my lab. Dr. Vedanayagam, by the way, recently moved to a faculty position at the University of Texas at San Antonio, where his new lab will continue digging deeper into these topics. In addition, we benefited from several collaborations, and I’d like to highlight key contributions from members of Benjamin Loppin and Raphaelle Dubruille’s group at the University of Lyon, France, and from Colin Meiklejohn’s lab at the University of Nebraska.
Our two new PLOS papers broaden our recent research that appeared in Developmental Cell and Nature Ecology & Evolution, and unveil and unravel these genetic mysteries in the Drosophila simulans model.
One of our papers shows that a network of X-linked genes of the Distorter on the X, or Dox, family are suppressed by multiple siRNA genes. And if we allow these selfish genes to be fully active, they cause terrible consequences, including elimination of sons and/or complete male sterility. In a very telling set of experiments, it turns out that male flies that lack these critical siRNA protectors are just fine if we also remove their selfish target genes on the X chromosome. This tells us that all of these are useless battles and that none of these genes are intrinsically needed for reproduction.
The other paper generalizes our findings, by discovering many previously unknown genes that are silenced by the RNA interference (RNAi) pathway. We are very excited by these findings, as they suggest RNAi loci may broadly pinpoint otherwise hidden conflicts within genomes. Interestingly, many of these involve very recently evolved genes with potential roles in sex chromosome battles. We hope to test some of these in the future.
Overall, these are striking examples of genetic pathways that are important for just a blip of evolutionary time, because they are born out of this period of conflict. Things calm down over time as the problematic genes get mutated or lost. Such fast evolution in response to an internal genomic conflict is actually a great example of the Red Queen hypothesis. This was proposed in 1973 by Leigh Van Valen, as inspired by Lewis Carroll’s tale Through the Looking-Glass. In one scene, the Red Queen says to Alice: “Now, here, you see, it takes all the running you can do, to keep in the same place.” In our scenario, it might seem that having equal numbers of boys and girls is a default outcome. However, it turns out that evolution can run at breakneck speed simply to maintain the status quo — in this case, deviating from, and quickly returning back to, an even sex ratio in the population. That’s why these types of selfish genes are so difficult to find — when the suppressors are working well, then there are no abnormalities to see. We often don’t appreciate when things seem perfectly normal — until things go awry.
Why is this developmental biology research being done at a cancer center?
The Sloan Kettering Institute and MSK have a long history of what we call discovery science, fundamental research, or sometimes basic research. It’s a commitment to pursuing a deeper understanding of biological functions and mechanisms, without a specific application in mind. For example, the new frontiers in cancer treatment, like immunotherapy and cancer vaccines, are only possible thanks to decades of research into foundational questions in biology.
We never know where fundamental research can lead. For example, the initial work that uncovered the RNAi/siRNA pathway itself was conducted by Andy Fire and Craig Mello using the soil worm C. elegans. The RNAi technique revolutionized genetics and was recognized by their Nobel Prize in 2006, and more recently led to a new class of siRNA drugs. Our current research now provides insight into one of the most critical biological processes that depends upon RNAi and siRNAs.
Regarding the implications of our findings on selfish genes in, say, humans, we won’t know until further work is done. Sex chromosomes are locked into long-standing genetic conflicts, even in mammals, and so the principles we are uncovering might be relevant to human infertility. Alternatively, since cancer cells exploit genetic alterations to gain advantages, it’s possible that molecular strategies of selfish genes might inform principles that operate in cancer. In the meantime, we remain excited about how fundamental research in fruit flies continues to add to the sum of human knowledge on biology and life.