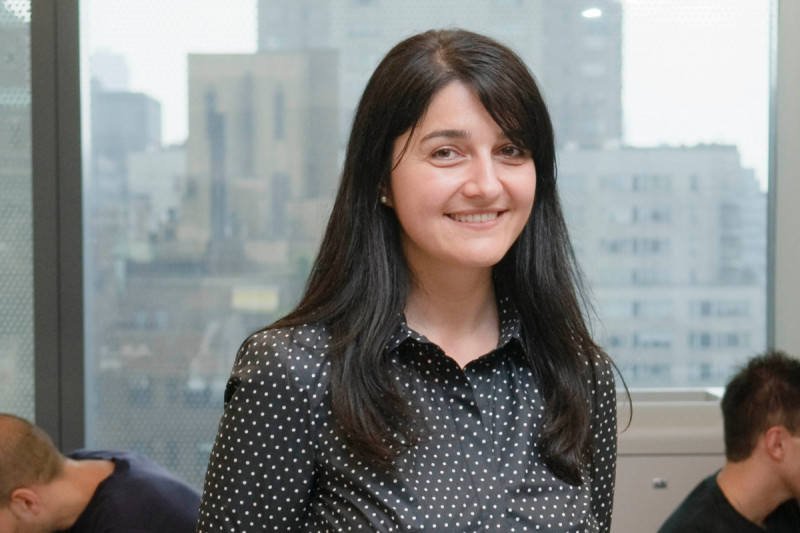
Molecular biologist Christine Mayr studies how the noncoding parts of transcription units (3’UTRs) determine protein function and protein localization. We spoke with Dr. Mayr soon after she joined the Sloan Kettering Institute’s Cancer Biology & Genetics Program in 2009.
My interest in science started when I was a child growing up in the area around Munich, Germany. I was given a book about the solar system, which I found so fascinating that I wanted to become an astronaut when I was older. I also got into the science-fiction television show “Captain Future,” shown only in Germany and Japan. It featured an astronaut who travels around the universe discovering new worlds. But I realized that I was probably born about 1,000 years too early to be doing that sort of work as an astronaut, so I knew I had better make another career choice.
Abandoning my goals of exploring space, I did maintain my interest in astronomy and particle physics. Already as a teenager, I was totally certain that I was going to be a physicist. In my first year in college, at Ludwig-Maximilians University, in Munich, I studied physics but very quickly realized it was not for me.
At that time a lot of my friends wanted to go to medical school and somehow I convinced myself that I also wanted to do this. We started by studying the basics, chemistry and biochemistry, which I loved. And I was also fascinated by how a disease develops. By the third year of the program, I realized that I may be better suited for a lab, where I could focus on the mechanism behind the disease.
At that point I thought that my real interests lay in biochemistry, but I had already started several things so I decided to finish medical school. I ended up doing my residency in hematology/oncology, and it turned out that I liked it much more than I had initially expected.
I still knew that what I really wanted to pursue was basic research in a laboratory. After having obtained a PhD in Immunology at Humboldt University, in Berlin, I was interested in tumor immunology. At the time, people were theorizing that the immune system might be trained to fight against cancer, in a field known as immunotherapy. I found it fascinating that cancer cells are most of the time invisible to the immune system, meaning that they had some mechanism by which they could hide from its destructive capacity.
I did my post-doctoral fellowship in Dr. Michael Hallek’s laboratory, back at Ludwig-Maximilians University. In the lab, we stimulated the tumor cells in order for them to become recognizable by the patient’s T cells. Specifically, we were able to generate and characterize an antigen-specific immune response against chronic lymphocytic leukemia (CLL) cells.
Identifying Prognostic Factors for Chronic Lymphocytic Leukemia
We also applied our stimulation techniques to do cytogenetic analyses in CLL. In order to find new chromosomal aberrations that may lead to cancer, you need dividing cells, known as metaphases. But the CLL cells do not divide in culture (growing cells in a laboratory setting), therefore it was very hard to do metaphase cytogenetic analyses.
However, with our stimulation technique we were able to generate metaphases in culture. We found that, contrary to popular belief at the time, a significant percentage of patients with CLL have chromosomal translocations — chromosomal abnormalities that occur when pieces of DNA are exchanged between two chromosomes. We were also able to define the occurrence of chromosomal translocations as a new prognostic factor in CLL.
This is important because some people with the disease were dying within two years of diagnosis, while others could live for another 25 years. Developing good prognostic factors to distinguish between the two groups to help create treatment plans was a significant step forward.
But as it often is the case with my interests, once I had some success with one area of interest, I grew bored of it and needed to move onto something else. Around this time, in 2004, I was at a conference where I heard a speaker talking about microRNAs (miRNAs), a subject with which I was completely unfamiliar (miRNAs are short pieces of RNA that do not encode a protein but do control the expression of genes).
Listening to the talk, I became fascinated by what essentially was a new gene regulatory mechanism that affects plants, fungi, and animals, including humans. They were first discovered in 1993, but no one knew that they were so ubiquitous until 2001. I became instantly hooked.
Knowing that I wanted to do my next postdoc fellowship in the US, I applied to labs that were working on miRNAs. At the time, there were only a handful of such labs, one of the biggest of which was David Bartel’s laboratory at M.I.T.’s Whitehead Institute for Biomedical Research. I was lucky enough to be accepted into David’s lab in 2005. In his lab, everyone studied miRNAs in different organisms, including worms, flies, mice, frogs, and plants. Because of my background and my interest in cancer, I became the person studying them in humans.
miRNAs and Cancer
At the time, there was almost nothing known about the role of miRNAs in cancer. Researchers began by looking to see if there was a change in miRNA expression levels between normal tissue and cancer tissue and then to find out what a specific miRNA was doing. But every miRNA was predicted to have hundreds of target genes, so in the end it was difficult to find out which gene was responsible for the observed effect. As a consequence, I took an alternative approach by looking at it the other way around — starting with the target genes.
An mRNA has three basic parts. The first, known as the 5’UTR, is the beginning section. It includes the promoter component that switches the gene “on” and “off.” The middle section codes for the protein that is responsible for the function of the gene. And the end section, the 3’UTR, is where miRNAs bind. The binding of miRNAs to the mRNA leads to downregulation of gene expression.
I thought that if the 3’UTR of an mRNA was missing, the miRNA would not be able to bind, which would mean that the gene is no longer regulated by the miRNA, and its expression should increase. Therefore, I was looking for an mRNA that was regulated by miRNAs and that had lost its 3’UTR in cancer and I found a gene called HMGA2.
HMGA2 is regulated by the let-7 family of miRNAs, which has multiple binding sites in the 3’UTR of the HMGA2 transcript. These binding sites are lost due to chromosomal translocations in a wide variety of human tumors including lipomas and sarcomas. To prove that loss of regulation by let-7 was responsible for the overexpression of HMGA2 in cancer, I introduced point mutations where let-7 was supposed to bind to prevent let-7 from downregulating HMGA2. The end result was that normal cells became transformed into cancer cells.
Therefore, HMGA2 was the first example of an oncogene being overexpressed due to loss of its miRNA binding sites, and we had identified a new mechanism for the development of cancer. (An oncogene is a gene that, when mutated or expressed at high levels, helps turn a normal cell into a cancer cell.)
Alternative Cleavage and Polyadenylation
In the case of HMGA2, the 3’UTR was lost in cancer cells because of a chromosomal translocation. The next thing I was interested in learning was if there were other ways for a cancer cell to lose the 3’UTR of oncogenes (and to escape from regulation by miRNAs). By searching the literature, I found another example for loss of the 3’UTR in a cancer known as mantle cell lymphoma. The 3’UTR of an important gene was missing through a mechanism other than chromosomal translocations in 20 percent of patients — and these were precisely the patients who had a more aggressive form of the disease.
So here you had one gene and one disease, but I thought that loss of the 3’UTR was probably much more widespread in cancer because loss of the 3’UTR provided an advantage for this tumor. The question now was, how widespread is this, and is this happening in all cancer types?
This is how I got interested in alternative cleavage and polyadenylation. At the end of every mRNA there is a signal that indicates that the end of the mRNA is reached (the polyadenylation signal). I found that many of the genes I Iooked at had these signals (or variants of this signal) also in the beginning of the 3’UTR. And it turned out that these signals that were located in the beginning of the 3’UTR (we call them alternative polyadenylation signals) were used in cancer cells.
Therefore, cancer cells express shorter mRNAs in addition to the full-length mRNAs that are expressed in normal cells. In most of the cases, over 90 percent of the 3’UTR was gone by using the alternative polyadenylation signals. This was true for nearly half of the genes I investigated and it was true for all cancer types I looked at. So, I think that this is a pretty widespread phenomenon.
The next question was what might be the functional consequence of having such a short 3’UTR? And I found that there is much more protein being made from the mRNA with the shorter 3’UTR than with the longer 3’UTR. This difference in protein expression was so dramatic that expression of the short form of the oncogene IMP-1 was sufficient to transform normal cells into cancer cells, whereas the expression of the long form (the form that is expressed in normal cells) had no such effect. This means that alternative polyadenylation is a new and widespread mechanism for cancer cells to overexpress oncogenes.
The new question became how is this happening?
A Lab of Her Own at Sloan Kettering Institute
Wanting to start my own lab to answer this and other questions, I decided to come to Sloan Kettering Institute (SKI) at Memorial Sloan Kettering Cancer Center. Although I routinely change the subjects I study, the one constant through all my work has been my interest in cancer biology. It was very important to me that SKI is expanding and growing in so many interesting ways, bringing in a whole host of young researchers, each one doing fascinating and cutting edge work. I knew I wanted to be a part of that with my own work.
My research has found that miRNA regulation might be lost in a number of ways, and we showed that one mechanism is alternative cleavage and polyadenylation. Recognition of different polyadenylation sites can lead to changes in 3’UTR length with a loss (or gain) of regulatory sequences, thereby regulating gene expression during cancer development.
Recently, it has been shown by others that shortening or lengthening of 3’UTRs can be detected in diverse biological processes such as T cell proliferation, neuronal activation and differentiation during embryonic development. It seems that proliferating, immature, and transformed cells have mRNAs with shorter 3’UTRs, whereas terminally differentiated cells mostly have mRNAs with full-length 3’UTRs allowing a tighter regulation.
What is interesting to me now is the question of why the same proximal polyadenylation signal that is present in normal cells is being ignored in normal cells but recognized and used in cancer cells. I want to find the mechanism that explains this. To do so, I will go off into new directions, away from miRNAs. It will be a challenge, but if we find out the mechanism behind this, we might be able to control it and potentially open up new ways to treat cancer.