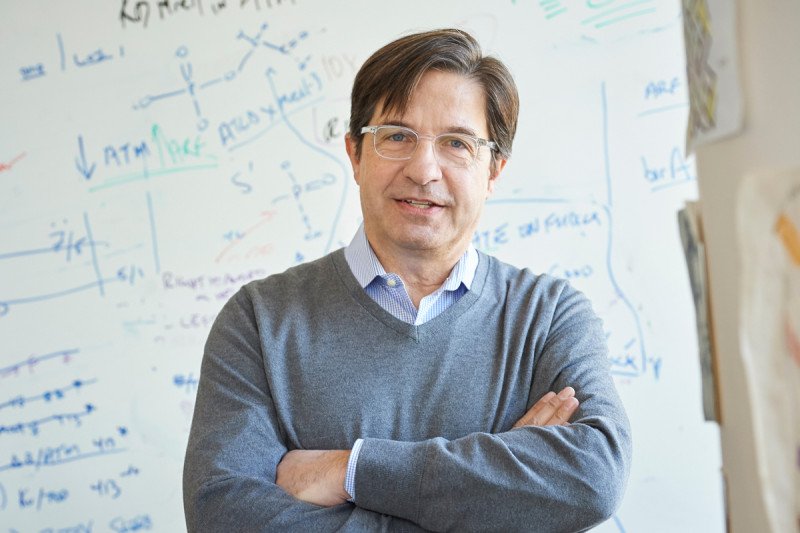
John H.J. Petrini, Molecular Biologist -- "It's been gratifying to know that our work is contributing to the understanding of chromosome maintenance and the processes that predispose people to cancer."
Molecular biologist John Petrini investigates the repair of chromosomal breaks and the activation of the DNA-damage-induced cell-cycle checkpoints. We spoke with Dr. Petrini in 2005, soon after he became the incumbent of the Paul A. Marks Chair in Molecular Biology.
As an undergraduate chemistry major at Kalamazoo College in Michigan, I was more interested in music than science. I do, however, remember being intrigued by the evolving study of recombinant DNA at that time — the early 1980s — and even pursued a related science project at the University of Michigan. But at the time, I preferred playing guitar. It wasn’t until later that I really got hooked on science.
After graduation, my band mates and I traveled to California in hopes of performing professionally. We did survive a few months out there. But when I realized we were making more money doing lawn work than playing music, I knew it was time for a change. So I went back to the University of Michigan for graduate school. Even though I consider myself a geneticist, the only genetics course I’ve ever had — a course in bacterial genetics — was at Michigan. It had a big impact on how I think about our work.
Fascinated by Gene Rearrangement
I then pursued postdoctoral work at Fox Chase Cancer Center in Philadelphia. During this time, I became fascinated by the emerging view that even though there were highly organized processes in cells and specialized venues for the rearrangement of chromosomes, changes in genetic material were largely governed by generic factors, with help from unknown cell-type-specific “magic stuff.” Personally, I was interested in the generic stuff, as it was likely to be universally important.
After a year in Philadelphia, I moved to the Dana-Farber Cancer Institute in Boston, where my studies of gene rearrangement began in earnest. I learned a great deal about this process from yeast, an organism often studied as a model emulating cells in mammals such as humans. It was the late 1980s. Scientists were just beginning to understand DNA damage and its possible role in cancer.
The prevailing opinion at the time was that chromosome instability — particularly an inability to repair damaged DNA — was sufficient to initiate cancer development. I believed that this was somewhat accurate. But I’m rather stubborn and have a sort of knee-jerk suspicion of such facile explanations — the natural skepticism of a scientist, I guess. I thought there had to be more to it. In yeast, chromosome instability causes the organism to die. So how can damaged DNA be enough to cause cancer? I then began testing whether or not this dogma was true. In 1994, I moved to the University of Wisconsin, Madison, and I came to the Sloan Kettering Institute in 2001.
The Importance of Rad50
Today we know that problems in maintaining chromosome integrity are part of the cancer process, but not the whole story. In my laboratory, we’ve characterized a complex of three proteins that are essential for DNA repair to occur: Mre11, Rad50, and Nbs1. Together they make up the Mre11 complex, which senses broken chromosomes. We’ve shown that mutations in the Mre11 and Nbs1 genes are responsible for two rare genetic syndromes that confer a greatly elevated risk of cancer, especially leukemias and lymphomas.
In 2002 we were part of a multi-institution project suggesting that the Rad50 protein has a “zinc hook” that acts like Velcro® to bring together the various participants in the DNA repair process. Earlier this year, we used yeast to prove that hypothesis and showed that without a functional Rad50 protein, DNA repair cannot occur.
We also do a lot of work in mice. For example, we developed a mouse model with a mutated Rad50 gene, which caused a total depletion of bone marrow cells in the mice by the time they were two months old and predisposed them to lymphoma. This finding and our other studies in mice have really reshaped our thinking about how this all works — how DNA breaks are detected, activating other parts of the process — and above all, how, in a complex animal with multiple organ systems, disruption of these basic processes plays out in the form of disease. We’re continuing this work to gain a better understanding of the role of DNA damage in cancer initiation and progression.
It’s been gratifying to know that our work is contributing to the understanding of chromosome maintenance and the processes that predispose people to cancer. I’ve also been incredibly fortunate to have great people in my laboratory. I look forward to seeing them go on to run their own labs — and some day, for the people in their labs to become my “scientific grandchildren.”
Coming to the Sloan Kettering Institute was very liberating for me. This institution has excellent scientific colleagues and resources, all of which facilitate the work we do, empowering us to move forward. Science is hard, but it’s great to be in a place where we can do it a little more easily.