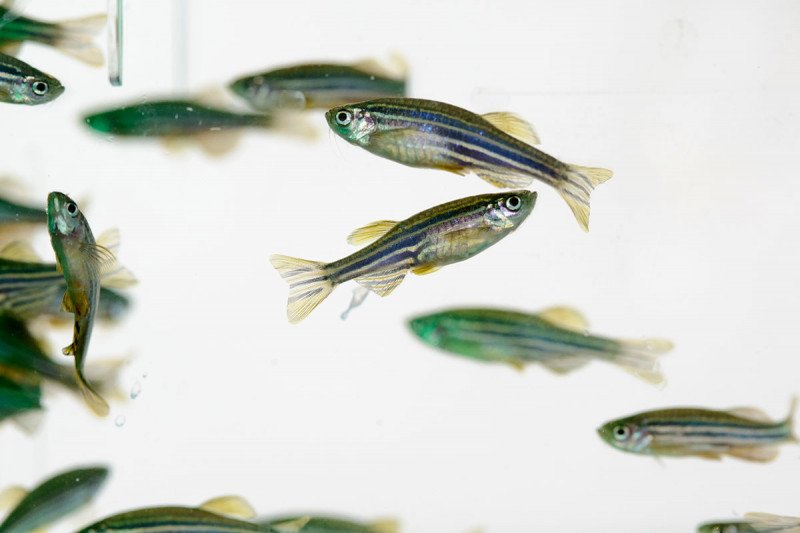
The zebrafish (Danio rerio) is a useful model organism for studying development and cancer.
If you’re a zebra, you want your stripes in order. Come to the savannah wearing spots and someone might mistake you for a leopard.
Proper pigmentation is important for a variety of animal endeavors, including finding and selecting a mate, hiding from predators, and protecting oneself from damaging UV light. But it’s even more than that.
“Pattern formation in the embryo is how every animal organizes its tissues,” says Richard White, a physician-scientist in the Cancer Biology and Genetics Program at the Sloan Kettering Institute. “That’s true of the pancreas, it’s true of the kidney, it’s true of the skin. Patterning is how animal bodies are made.”
How the Richard White Lab Studies Zebrafish to Understand Cancer
Dr. White’s lab studies patterning in an animal called the zebrafish, a small freshwater fish with distinctive black-and-white stripes running down its length.
In a new study, published today in the journal Developmental Cell, Dr. White and graduate student Yan Zhang reveal how zebrafish get their stripes. Unexpectedly, it has to do with insulin.
“That was really a surprise to us,” Dr. White says. “We generally think of insulin as the sugar hormone. Nobody expected it to play a role in skin pigmentation.”
More than just a curious finding from the aquarium, the result has relevance to human cancer.
Making a Stripe
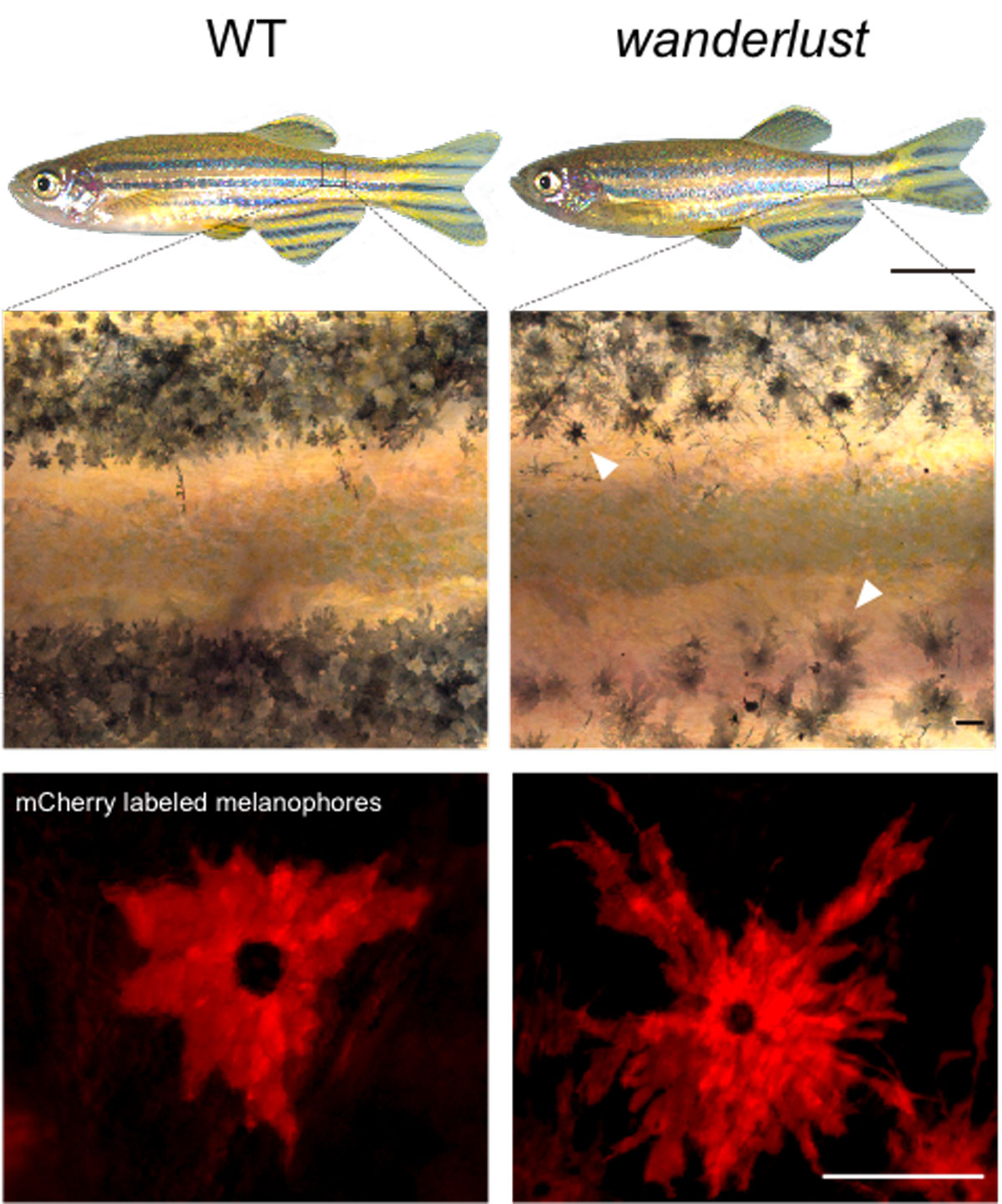
A normal (WT) zebrafish has crisp stripes and melanophores with a moderate number of protrusions called dendrites. The wanderlust zebrafish has blurry stripes and melanophores that are hyper-dendritic.
For a stripe to form in a fish, the cells that make melanin (called melanophores) have to line up in the right place in the fish body, spaced a certain distance apart from the melanophores in a neighboring stripe. If a stray melanophore ends up in the valley between, then you’ve got more of a polka dot situation than a stripe on your fins.
Several years ago, he and his team identified a mutant fish that had wayward melanophores, leading to fuzzy stripes. They called it wanderlust.
To explore what signals cause the wanderlust phenotype, Dr. White’s team used a method called chemical screening to test a large number of compounds to see whether any could “rescue” the mutant — make it appear normal again. They put the fish in 96-well plates and then added each of the chemicals to the water.
Among 1,280 chemicals they screened, four were able to rescue the mutants. To the team’s surprise and delight, all four chemicals blocked the same pathway — the one that starts with insulin.
“We couldn’t have asked for a better result,” Dr. White says. “I didn’t believe it at first, to be honest.”
From previous studies, Dr. White’s team knew that wanderlust fish have a mutation in a gene called Bace2. In this study, they show that the one of the normal functions of the Bace2 protein is to cleave the receptor for the hormone insulin. This cleaving effectively shuts down the signal from this hormone. In the mutated form, Bace2 no longer cleaves the insulin receptor and the signal stays on.
Melanophores receiving this continuous insulin signal don’t know when to stop growing and migrating. They divide excessively, wander around, and end up depositing melanin in the wrong places.
Mixed Signals
According to Dr. White, the results offer a clear example of how a long-range signal — such as a hormone — can interact with local factors to produce a characteristic pattern.
“It’s been known for a long time that even an early embryo produces hormones,” he says. “Some of these hormones support growth in an undifferentiated way — like giving it gas.”
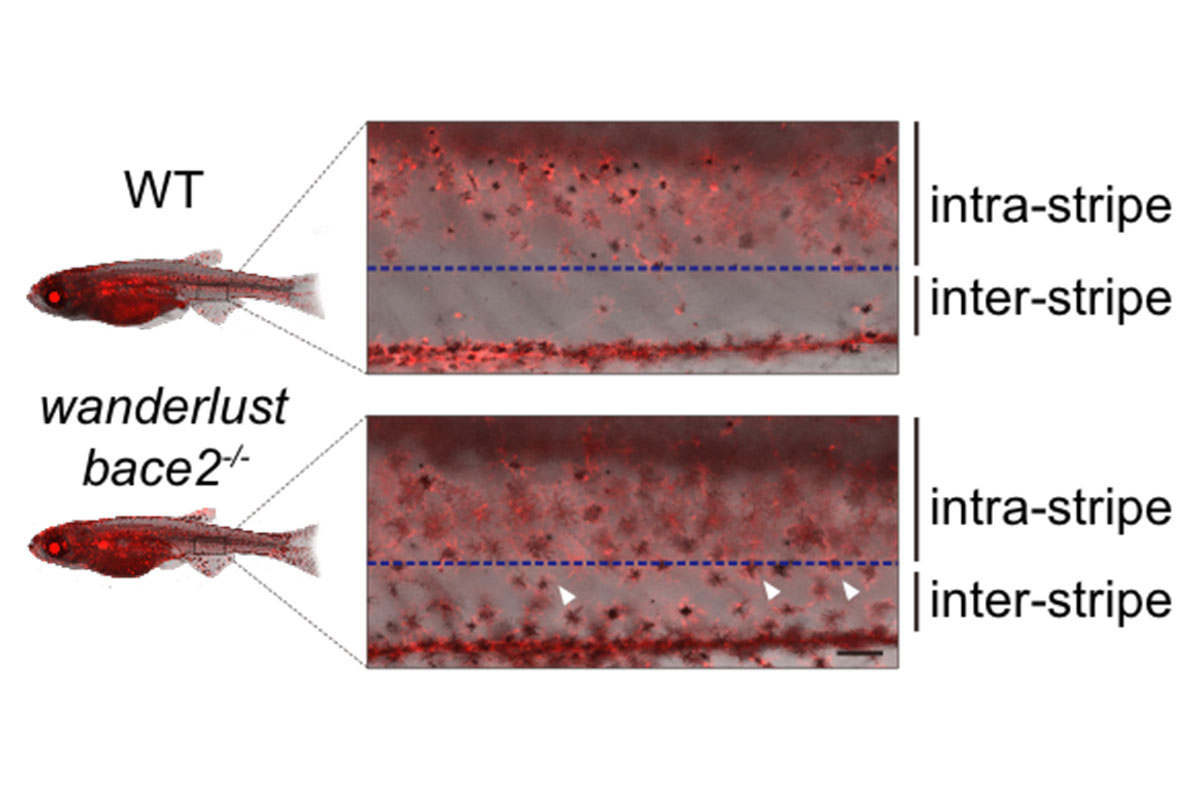
In the wanderlust fish, more melanophores are found in the white space between stripes.
But not every tissue responds to a given hormone in the same way. A hormone like estrogen, for example, doesn’t work on every cell in the body. And the same is true in developing embryos.
“Each cell needs to decide how it’s going to respond to that very general factor in order to pattern itself correctly,” says Dr. White. “Otherwise, it’s just pressing on the gas pedal without any brakes. How would the cell know when to stop?”
By cleaving their insulin receptor, pigment cells know to stay in place.
Color and Cancer
The new results could help explain some curious findings from the clinic. People with a rare disease of excess insulin secretion called acanthosis nigricans sometimes develop striking changes in skin pigmentation. In humans, melanin is produced by cells called melanocytes, equivalent to fish melanophores. Might a mechanism similar to the one described here for fish melanophores play a role in humans? “It’s an interesting idea,” Dr. White says. “But we haven’t shown that directly in the paper.”
More practically, there could be an important link to cancer. Anything that decreases the function of Bace2 could result in heightened activity of the signaling pathway activated by insulin, called the PI3K/mTOR pathway. Mutations in this pathway are already known to be involved in cancers like melanoma, which are derived from the melanocytes. Perhaps another way that these cells become cancerous is by altering the expression of Bace2.
“It’s a question many of us are interested in — how do things that happen in a fish embryo relate to what happens in adults with cancer? We’re actively studying this connection, and should have more to report soon,” Dr. White says.